Research
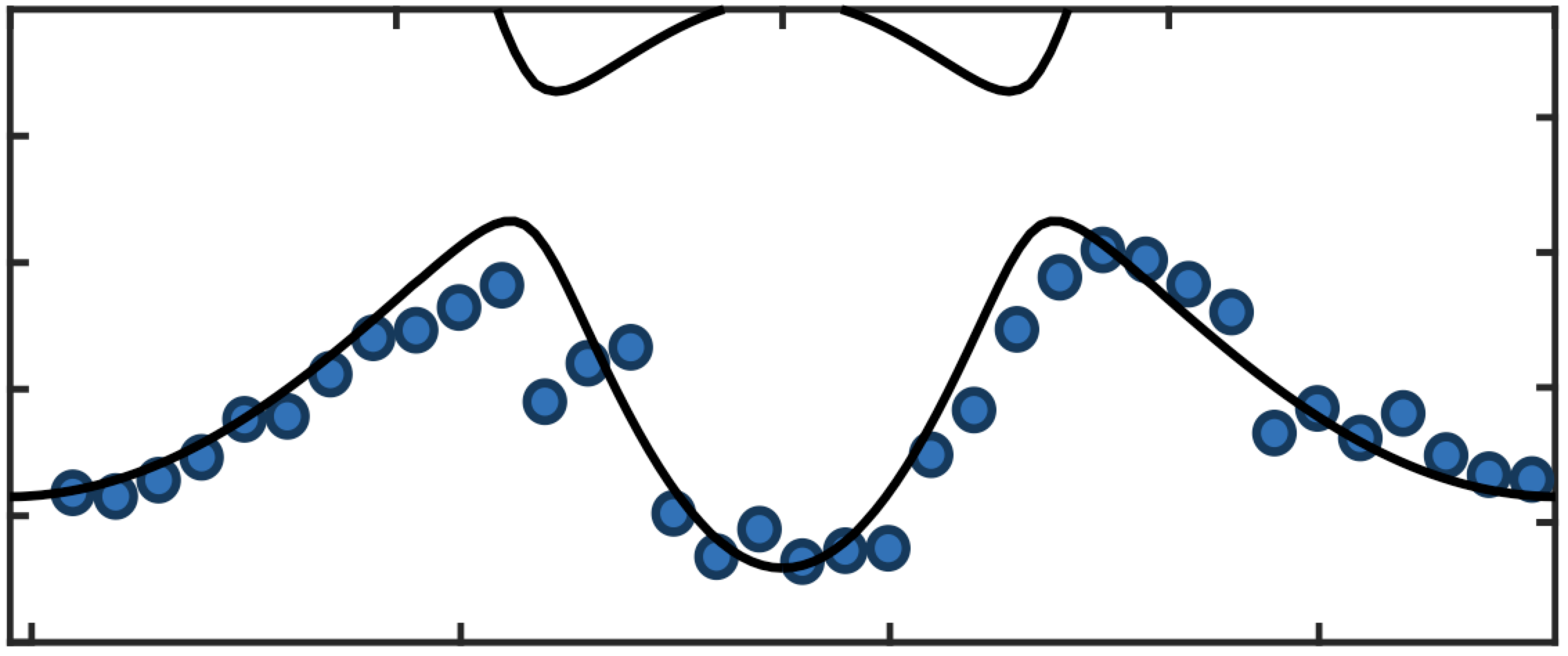
Nonequilibrium Dynamics
Predicting and controlling the behavior of non-equilibrium many-body quantum systems is a major challenge of modern physics. In addition to their fundamental importance for quantum statistical physics, advances in this area have potential applications ranging from materials science to quantum information. Due to their experimentally accessible timescales and exquisite spatiotemporal controllability, trapped ultracold atomic gases are an ideal tool for studying many-body non-equilibrium quantum dynamics. Experiments in our group study a wide variety of dynamical phenomena, from Floquet-Bloch bands to quantum thermodynamic engines.

Quantum Interactive Dynamics
Hybrid quantum-classical systems enable a new kind of dynamics, involving repeated observation of an evolving quantum system followed by feedback conditioned on the measurement outcomes. While much effort in this area has focused on preserving quantum information in a quantum computer, the guiding principles that underlie the use of dynamical evolution, measurements, and feedback to create structured "quantum interactive matter" are largely unexplored. Can we harness the counter-intuitive features of quantum measurement to create new states of nonequilibrium matter? What new material properties and capabilities are possible in the unexplored regimes of interactive quantum phases? Enabled by recent theoretical and experimental developments, our group is collaborating with the groups of Andrew Jayich, Matthew Fisher, and Sagar Vijay to investigate quantum interactive matter using a new potassium-based optical tweezer apparatus.

Quantum Quasicrystals
Quasiperiodicity has a profound impact on electronic structure, lying at the heart of phenomena ranging from quasicrystals to the quantum Hall effect to twistronics. Using degenerate quantum gases in quasiperiodic optical traps, our group explores the fascinating effects of quasiperiodicity on the static and dynamic properties of quantum matter.
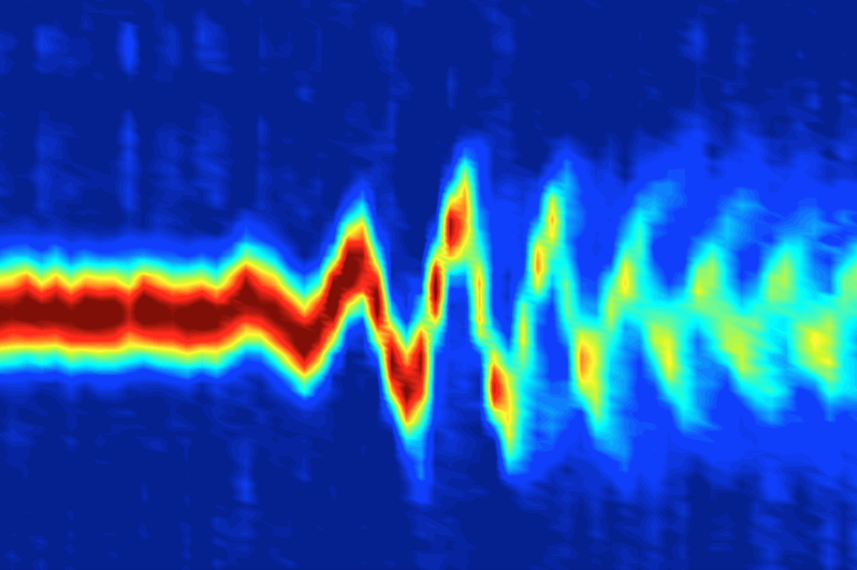
Quantum Simulation of Ultrafast Phenomena
we have built a unique physical quantum simulator of ultrafast phenomena, in which time-varying forces on neutral atoms in a tunable optical trap emulate the electric fields of a pulsed laser acting on electrons or nuclei in a binding potential. The simulator operates in regimes equivalent to those of ultrafast and strong-field pulsed-laser experiments, opening up a hitherto unexplored application of quantum simulation techniques and a complementary path towards investigating open questions in ultrafast science. Counter-intuitively, this approach emulates some of the fastest processes in atomic physics with some of the slowest.

Quantum Interfaces
A central challenge for the realization of useful quantum technologies is that the interfaces needed to control and functionalize quantum systems are unavoidably accompanied by surface-mediated decoherence, a poorly understood but ubiquitous process. Together with the groups of Ania Bleszynski Jayich and Kunal Mukherjee at Stanford and Norm Yao at Harvard, we are pursuing a new experimental approach to this fundamental challenge by investigating controllable coupling of delta-doped nitrogen-vacancy centers in diamond to specific atomic adsorbates on an engineered quantum surface.